Nuclear power should be a powerful ally in the fight against climate change but cost and safety issues hold it back. Could a new generation be safer and cheaper? Tom Heap meets the team behind the molten salt reactor that can use nuclear waste as fuel and is claimed to be significantly cheaper and safer than current reactors.
Ian Scott was a senior scientist at Unilever, pioneering research into skin-ageing, but when he retired from the field of biological sciences he became fascinated by the costs of nuclear power. Why had nuclear electricity - which we'd once been promised would be 'too cheap to meter' - become one of the most expensive forms of energy generation? The answer lay with the safety mechanisms that have to be built-in to reduce the risk of another Chernobyl or Fukushima. If he could develop a system that would be much safer then it would, almost certainly, be much cheaper.
Ian's central idea - to use molten salt as a coolant rather than water - caught the eye of energy authorities in Canada and Ian's company, Moltex, has plans to build its first reactor in New Brunswick. Significant safety concerns remain, with some in Canada concerned about Moltex plans to use spent fuel from conventional reactors and others raising fundamental issues about the corrosive qualities of molten salt and the generation of radioactive tritium.
Tom visits the Moltex laboratories and climate scientist, Tamsin Edwards, gauges the potential impact on greenhouse gas emissions.
What our experts say
We asked Society Fellows Professor Ian Farnan and Dr Eugene Shwageraus from the University of Cambridge to offer some observations on the potential of molten salt reactors (and other innovative nuclear fission reactors) in reducing carbon emissions if adopted on a mass scale. Their points take some of the themes of the programme a step further.
Professor Ian Farnan
One of the issues is ‘nuclear fade’: if the large number of reactors built in the 70s and 80s are not life-extended, any new build nuclear carbon avoidance may just replace those reactors (average reactor age in USA is 39 years).
One limiting economic factor for electricity generation by smaller units (100-300 megawatts (MW)) is that for smaller reactors to be competitive with a large reactor, 10-20 would need to be built to overcome the economies of scale of the larger reactors. The investment decision is shifted from the reactor site to the factory manufacturing the units and the order book required to make a final investment decision (FID).
Dr Eugene Shwageraus
The most significant challenge of molten salt reactors (MSRs) is monitoring and controlling the chemical environment at the interface between coolant/fuel salts and reactor structural materials. The local chemical environment may vary widely as a result of different and continuously evolving chemical species in the salt, temperature and temperature gradients, and salt flow velocity, as well as the type and intensity of radiation field. These variations can impact the rate of structural materials corrosion and other types of degradation, impacting the longevity of the components in the reactor. Physical separation of fuel and coolant salts (such as the Moltex design) can substantially alleviate this problem because the coolant salt's chemical purity is much easier to control than in traditional molten salt reactor where the salt contains a complex mix of nuclear fuel fission and transmutation products.
A high melting point of fuel/coolant salts also complicates the components inspection, maintenance and repair because all of these operations have to be performed remotely, at high temperatures and in a strong radiation field. These technologies do not currently exist and will have to be developed rather than borrowed from existing reactor types.
Finally, most promising molten salt reactor designs use lithium containing salts which, under neutron irradiation, would produce significant quantities of radioactive hydrogen isotope – tritium. At relevant operating temperatures, tritium rapidly diffuses through practically all common structural materials (e.g. stainless steels). Therefore, the salt needs to be constantly cleaned up to avoid radioactive contamination of the environment immediately surrounding the primary coolant circuit.
What are the co-benefits?
Professor Ian Farnan
The co-benefits may be the main benefits of MSRs. Rather than connect to the grid, they can serve as site specific electricity and energy sources. Hence, they can serve remote communities (thus the Canadian interest).
They can also have standalone applications in decarbonising the industrial sector (~20% of emissions). Due to their excellent thermal properties, molten salts increase the efficiency of electricity generation up to about 50%. The heat (550-650˚C) can be stored or used in many processes that are energy and/or electricity intensive. The cost of electricity from mass-produced MSRs touted to be up to $50 per megawatt hour would bring the provision of zero-carbon electricity or hydrogen production into play in industrial processes (beating out carbon capture).
Dr Eugene Shwageraus
Molten salt reactors can deliver heat at a high average temperature. This allows for:
-
Increasing thermodynamic efficiency of power conversion from current typical 33% to close to 50% using advanced working fluids.
-
More convenient opportunities for thermal energy storage, and therefore integration with intermittent renewables.
-
Heat to be provided to industrial customers, supporting a wider range of industrial processes.
-
Transportation of heat over longer distances to industrial and domestic heating purposes.
-
These are generally harder or impossible to achieve using conventional Light Water Reactors (e.g. European Pressurised Water Reactor (EPR) at Hinckley Point C).
Unlike water, salts can exist in liquid form at high temperature without requiring to be pressurised. This substantially reduces engineering requirements on structural materials which no longer need to resist high mechanical stress in addition to high temperature. The absence of pressure force reduces the number of potential faults that need to be considered in a reactor safety case. Furthermore, volatile and biologically active fission products, such as caesium and iodine, are readily soluble in molten salts and do not represent a major concern in accidents as in light-water reactors (LWRs) where these elements make up most of the radioactive source.
Are there any potential negative impacts of this idea?
Professor Ian Farnan
The corrosiveness of molten salts should not be underestimated. While corrosion resistant alloys were developed at Oak Ridge in the prototypes and appeared to perform quite well, they only ran for a short while. Testing of materials, welding techniques etc. needs to take place. Any air leak into the system produces hydrogen fluoride which is even more corrosive. Any lithium in a reactor can be transmuted to tritium. Tritium can diffuse through nearly anything at high temperature and tritium extraction or control would be a regulatory concern. Finally, proliferation is always a concern.
Dr Eugene Shwageraus
Although the research and development effort required is substantial, so is the potential contribution of molten salt reactors to net-zero. The issues are relatively well-understood and promising ways of their mitigation are being actively studied at the moment. Molten salt reactors are comparable in terms of technological maturity to many other technologies being seriously considered currently in decarbonisation pathways. Molten salt-cooled reactors (such as Moltex) have a competitive edge because the coolant salt can be kept clean relatively easily to assure integrity of reactor structural components.
Further reading
-
Beerten, J., Laes, E., Meskens, G. and D’haeseleer, W. (2009) Greenhouse gas emissions in the nuclear life cycle: A balanced appraisal, Energy Policy, 37 (12), 5056-5068
-
Fratoni, M. (2021) Fluoride-Salt-Cooled High-Temperature Reactors, Encyclopedia of Nuclear Energy, 602-608
-
IEA (International Energy Agency) (2018) Data and Statistics 2018
-
Ignatiev, V. V. (2021) Molten Salt Reactors, Encyclopedia of Nuclear Energy, 553-568
-
IPCC (2014) Climate Change 2014: Mitigation of Climate Change. Contribution of Working Group III to the Fifth Assessment Report of the Intergovernmental Panel on Climate Change, Cambridge University Press
-
Krepel, J. and Kramer, K. J. (2021) Molten Chloride Fast Reactors (MCFRs), Encyclopedia of Nuclear Energy, 625-642
-
Roulstone, A. (2019) Meeting the UK’s 2050 De-carbonisation Targets for Electricity Generation: the contribution of Nuclear Energy. PhD thesis, University of Cambridge
About the series
39 Ways to Save the Planet is a new radio series by BBC Radio 4 developed in partnership with the Society and broadcast in 2021. It showcases 39 ideas to relieve the stress that climate change is placing on the Earth. In each 15 minute episode Tom Heap and Dr Tamsin Edwards meet the people behind a fresh and fascinating idea to cut the carbon.
Over the course of 2021, the Society will be producing events and digital content to accompany the series.
Episode 30: New nuclear
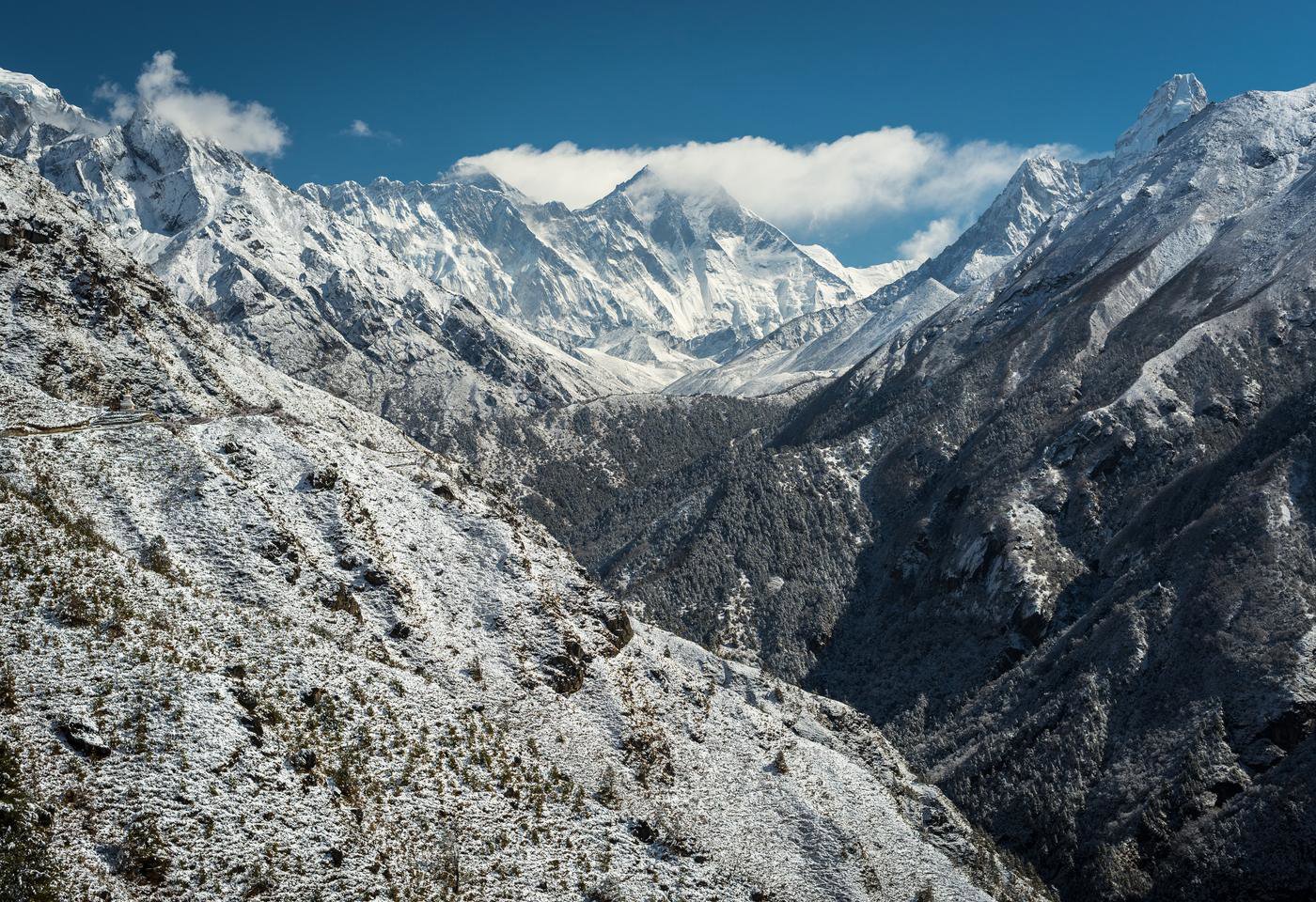
Featured card image: BBC
Featured banner image: Chalabal/AdobeStock